Spatial biology in drug discovery and development: opportunities and challenges
The recent panel discussion at the 3rd International Spatial Biology Congress provided valuable insights into the transformative potential of spatial biology and directions the field needs to move towards to integrate these technologies into drug discovery and development.
The advent of immuno-oncology has caused a shift from trying to find drugs that kill tumour cells, to a larger focus on harnessing the TME to fight cancer. In this scenario, it is crucial to be able to understand the TME and the interactions between different cell types and molecules within the TME and between TME and tumor cells. Spatial biology techniques, whose development couldn’t be more timely, helps in discovering and contextualizing these interactions, providing insights that were previously unattainable with traditional approaches due to lack of granularity (bulk RNA-seq) or lack of spatial information component (single-cell RNA-seq). But can they be used effectively to help bring new therapies to patients? And how?
Going from discovery to validation
Using genomic data to validate drug targets has been shown to reduce failure rates in clinical drug development. It’s no surprise that pharmaceutical companies have been embracing this approach increasingly in recent times. However, these traditional methods often rely on measuring averaged data across populations, which can obscure critical variations at the cellular level. Moreover, being limited to the presence or absence of a marker, they fail to consider how cells interact in situ thus providing a narrow understanding of disease mechanisms.
With spatial biology instead researchers can now observe how analytes (proteins, RNAs, metabolites, lipids) and cells behave in the TME in specific contexts (such as proximity to particular cell types, e.g. malignant cells), thereby opening a window into disease biology and providing stronger biological links for drugging candidate targets.
There is therefore a strong argument in favour of the integration of spatial data into existing pipelines for target identification and validation, which will also make it easier to assess treatment responses effectively.
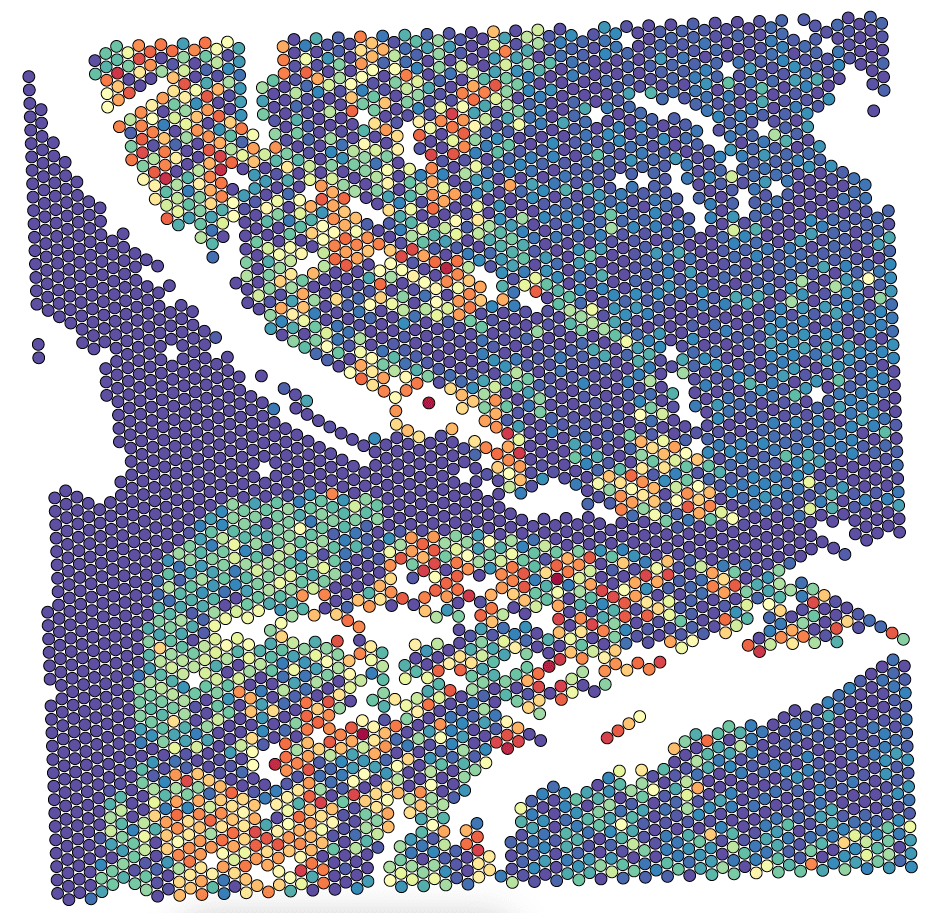
Adding the third dimension - and beyond
Spatial technologies are developing at a very fast pace. For example there is a race to increase resolution with some technology providers already offering solutions that are capable of measuring biomolecules at subcellular level. However, currently such technologies are using layers of tissue that are 5 micrometres thick, thereby missing a lot of three-dimensional information. To gain insights into the third dimension, researchers are using workarounds such as combining consecutive tissue sections along the z-axis but this presents a number of challenges from a computational and data analysis perspective.
There is a need to move towards being able to analyse thicker blocks of tissue, ideally up to 200 micrometers. This would allow scientists to gain more insights into the 3D structure of cells and tissues, build models to classify cells in their three-dimensional context, and answer questions like ‘where is the tumor starting to metastasize?’ to really dive deep into a disease’s biology.
Beyond that, adding time as the fourth dimension could be incredibly useful for target validation. Today’s technologies might not be mature enough yet, but one can imagine developing wet lab models that allow researchers to perturb biology and observe behaviour of cells and molecules, in real time, within the context of the tissue/disease microenvironment.
New research is coming online about the evolution of tumour cells and how they behave over time giving rise to tumour heterogeneity and developing drug resistance. Being able to take spatial biology measurements over time on ex-vivo tissue models is a very attractive prospect for scientists involved in designing new therapies. These models can include the tumour cells, stroma, immune cells and blood vessels, and really reflect the real composition of the tissue microenvironment. This would allow researchers to perturb the model with small molecules or activate gene constructs and measure the effect of the perturbation in real time to validate targets or other therapeutic hypotheses. From there, the next question would be - How do we convert this into high throughput platforms, so we can observe the effect of small molecules and test drugs at scale?
Challenges and practical considerations
The practical application of spatial technologies in pre-clinical and clinical settings remains an area of active exploration. While spatial techniques offer richer readouts compared to traditional methods, their high costs and complexity still pose significant barriers to widespread adoption.
In the context of drug discovery for example the number of identifiable potential drug targets is often prohibitively large to experimentally evaluate in spatial omics wet lab experiments. Instead of perturbing all 20,000 genes and observing what happens, a possible solution could be to first reduce the space to genes more likely to be involved in the biology of the mechanism being studied, and then perform spatial biology in the context of the real tissue.
Spatial technologies have the potential to revolutionize patient stratification by providing detailed insights into biomarkers and their spatial context. The complexity and heterogeneity of biological systems mean that a single biomarker might not be sufficient for optimal stratification. A multi-biomarker approach (to which spatial is particularly well suited) might be beneficial, but it must navigate the regulatory landscape to avoid prolonged approval times.
However for spatial omics to be implemented in clinical routine the costs need to come down, the technologies need to be simplified so that anyone could use them, and there needs to be a real effort toward reproducibility and reliability which at the moment is a challenge (groups cannot fully recapitulate results using different technologies). For this to happen there needs to be multidisciplinary collaboration amongst consortia, Dx/Cdx companies, clinicians, pathologists, and technology developers.
Another notable challenge is the harmonization of technologies. With the vast amounts of complex data that spatial technologies generate, there is a critical need for standardized analysis tools, file formats, and interoperable data platforms where analysis packages facilitate seamless data integration across the different technologies. This kind of standardization is crucial for translating spatial biology findings into clinical settings, where reproducibility and reliability are paramount, and consensus on experimental design and data processing protocols will help maximize the utility of spatial data, driving advancements in both research and clinical applications.
Conclusion
In conclusion, spatial biology holds immense promise for transforming drug discovery and development. Its ability to provide detailed, context-specific insights into cellular interactions and tissue environments marks a significant advancement over traditional methods. However, realizing its full potential requires overcoming challenges related to cost, data integration, and standardization. Moreover, by focusing on harmonizing technologies and developing robust, reproducible methods, the field can make significant strides towards clinical application, ultimately improving patient outcomes and advancing precision medicine.